By CLIFFORD K. HO April 20, 2013
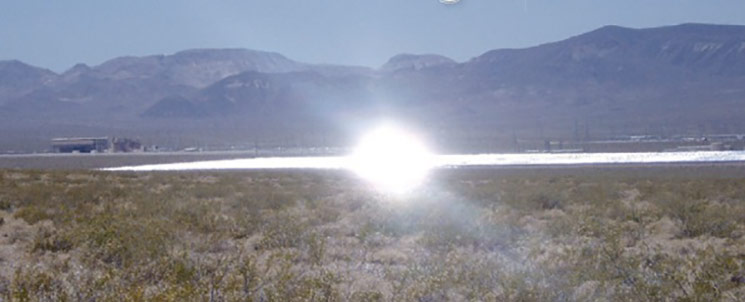
With growing numbers of solar energy installations around the world, solar glare is becoming an increasing concern. Impacts of glare, whether from photovoltaic (PV) or concentrating solar power installations, can range from discomfort to disability.

In 2012, CNN and local media reported that modules in a $3.5 million PV array on a parking garage at the Manchester-Boston Regional Airport had to be covered to alleviate glare to air-traffic controllers in the nearby control tower [1].
Options for mitigating these effects range from anti-reflective coatings and glass texturing for PV modules to blinds and screens, in certain situations. Perhaps the most effective method is through proper design and siting of the solar energy system, with consideration of its size, orientation, optical properties and location relative to key observation points. To assist with proper design and siting systems, solar developers have a few models and tools they can tap to evaluate the potential glare and ocular hazards [2, 3, 4].
The Effects of Glare

Glare from direct sunlight has been recognized for many years as a potential hazard for motorists and pilots [5-7]. Reports citing National Highway Traffic Safety Administration data estimate that solar glare causes nearly 200 fatalities and thousands of accidents involving motor vehicles each year, and the Federal Aviation Administration (FAA) reported that glare from direct sunlight contributed to nearly a dozen aviation accidents on average each year during an 11-year study [7].
While glare from direct sunlight is predictable — most problems occur during the mornings and evenings when the sun is close to the horizon — solar glare caused by reflections from solar energy installations can occur at varying times in unexpected locations. Glint (a momentary flash of light) and glare (a more continuous source of excessive brightness relative to the ambient lighting) can occur from various solar energy components such as PV modules, concentrating solar collectors/ mirrors and receivers.

Impacts of glint and glare on eyesight can include discomfort, disability, veiling effects, after-image and retinal burn [2, 5, 9-13]. Prolonged exposure to “discomfort glare” may lead to headaches and other physiological impacts, whereas “disability glare” immediately reduces visual performance. Disability glare can include after-image effects, flash blindness and veiling, such as that caused by solar glare on a windshield that might mask pedestrians or vehicles.
For the purposes of evaluating glint and glare from solar energy systems, colleagues and I [2] summarized the potential impacts to eyesight as a function of retinal irradiance (the solar flux entering the eye and reaching the retina) and subtended source angle (size of glare source divided by distance). The figure above shows the resulting “Ocular Hazard Plot” with three regions: (1) potential for permanent eye damage (retinal burn), (2) potential for temporary after-image, and (3) low potential for temporary after-image.
If the retinal irradiance or subtended angle is sufficiently large, permanent eye damage from retinal burn may occur (e.g., from concentrating mirrors). Below the retinal burn threshold, a region exists where a sufficiently high retinal irradiance may cause a temporary after-image, which is caused by bleaching (oversaturation) of the retinal visual pigments. The size and impact of the after-image in the field of view depends on the size of the subtended source angle. For a given retinal irradiance, smaller source angles yield smaller after-images, and the potential impact is less. Sufficiently low retinal irradiances and/or sub-tended angles of the glare source have a low potential for after-image and ocular impacts.
Factors that Impact Glare
A number of factors can affect both the intensity and perceived impact of glare: direct normal irradiance (DNI), reflectance, distance, size and orientation of the reflecting surfaces, and human factors. The DNI is the amount of solar irradiance striking a surface perpendicular to the sun’s rays. A typical clear sunny day may yield a DNI of ~1,000 watts per square meter at solar noon, with lower values in the mornings and evenings. The DNI provides the starting “strength” of the solar glare source, which can then be reduced by the reflectance of the PV module, mirror or receiver. The reflected light can be characterized as a combination of specular (mirror-like) and diffuse (scattered) reflections. Smooth surfaces such as mirrors and smooth glass produce more specular reflections with greater intensity and tighter beams (larger retinal irradiances and smaller subtended angles used in the figure above), while solar receivers, textured glass and anti- reflective coatings produce more diffuse reflections with lower solar intensities but greater subtended angles (see figure). The specular reflectance of mirrors can be greater than 90 percent, while the specular reflectance of PV glass can be as low 1 to 2 percent at near- normal incidence angles. However, at large (glancing) incidence angles (greater than 60 percent), the reflectance of PV glass can be 20 percent or more (even with texturing and anti-glare coatings).

The distance between the observer and the glare source can impact both the retinal irradiance and subtended source angle used in the figure. Atmospheric attenuation caused by particulates or humidity in the air will reduce the retinal irradiance with increasing distance. In addition, for a fixed size of the glare source, larger distances will typically yield smaller sub-tended angles of the glare source.(1)
(1) For flat specular surfaces, the subtended angle of solar glare remains constant until the distance increases to a point that the reflected glare image overfills the available surface area.

The size and orientation of reflective surfaces relative to the observer also impact the glare intensity and size. For example, a 3-kilowatt residential rooftop PV array will appear small relative to a 5-megawatt PV array at a given distance. The glare on the larger array can therefore grow to much larger sizes at longer distances than on the smaller array, yielding a greater potential for ocular hazards. Orientation of the array will also impact the effective viewable area, as well as the reflectance.
Finally, human factors such as ocular properties (pupil size, eye focal length, ocular transmittance) and light sensitivity will affect the retinal irradiance, subtended angle and perceived impact of the glare. Typical ocular properties for daylight adjusted eyes are provided in Ho, Ghanbari and Diver [2].
Mitigation Measures
Of the factors discussed above, those that can be controlled to mitigate the impacts of glare include reducing the specular reflectance and ensuring proper design and siting of solar energy installations. Textured glass and anti-reflective coatings can reduce the near-normal specular reflectance of PV modules to ~1 to 2 percent. The reduced reflectance and the increased scatter of the reflected beam can reduce the retinal irradiance and potential for ocular hazards.(2) However, reducing the reflectance is not always an option for some solar energy components, such as the mirrors for concentrating solar power collectors. Using screens and blinds to block glare is also possible, but it can be impractical or inconvenient for, say, air-traffic controllers needing to view the airport runways or for residents experiencing glare from a neighbor’s PV system.
(2) Increased scattering increases the observable size (subtended angle) of the glare image, which increases the potential for ocular hazards. However, the reduction in glare intensity and retinal irradiance from increased scattering and a larger subtended angle has a larger-order impact on reducing the ocular hazard.

Proper siting of solar energy installations, taking into account the size of the solar energy system, distance, orientation, environmental conditions and key observation points, is perhaps the most effective way to mitigate the negative impacts of glare. Models can assist in determining the impacts of glare (retinal irradiance, subtended angle and potential ocular hazard) from a variety of solar technology components that consist of flat, curved, specular and/or diffuse surfaces [2, 3].

In addition, Sandia National Laboratories has developed a web-based Solar Glare Hazard Analysis Tool (SGHAT) for PV systems that provides a quantified assessment of when and where glare will occur throughout the year, as well as potential effects on the human eye at locations where glare occurs [4].
SGHAT is free and available to the public at sandia.gov/glare.
The FAA has announced that it will disallow any new solar installations near airports without a quantitative glare analysis, including an assessment of visual impacts.
The SGHAT tool employs an interactive Google map where the user can quickly locate a site, draw an outline of the proposed PV array and specify observer locations or paths. Latitude, longitude and elevation are automatically recorded through the Google interface, providing necessary information for sun position and vector calculations. The user enters additional information regarding the orientation and tilt of the PV panels, reflectance, environment and ocular factors. If glare is found, the tool calculates the retinal irradiance and subtended angle of the glare source to predict potential ocular hazards shown in the figure. The results are presented in a plot that specifies when glare will occur throughout the year, with color codes indicating the potential ocular hazard. SGHAT also predicts relative energy production while evaluating alternative designs, layouts and locations to identify configurations that maximize energy production while mitigating the impacts of glare.
ABOUT THE AUTHOR
Clifford Ho (ckho@sandia.gov) is a distinguished member of the technical staff at Sandia National Laboratories, where he has worked since 1993 on problems involving energy, water and the environment. In the Concentrating Solar Technologies Department at Sandia, Ho has performed research on collector optics, solar glare, flux characterization, receiver design and modeling, and probabilistic systems analysis. He received his Ph.D. and master’s in mechanical engineering from the University of California at Berkeley in 1993 and 1990, and his B.S. in mechanical engineering from the University of Wisconsin-Madison in 1989.
ACKNOWLEDGMENTS
Sandia National Laboratories is a multi-program laboratory managed and operated by Sandia Corp., a wholly owned subsidiary of Lockheed Martin Corp., for the U.S. Department of Energy’s National Nuclear Security Administration under contract DE-AC04-94AL85000.
REFERENCES
[1] CNN, 2012, Solar Panels Cause Trouble at
Airport, Heather Hamel reporting, WMUR, Aug. 30, 2012; cnn.com/video/standard.html?hpt=hp_t3#/video/bestoftv/2012/09/01/nh-dnt-airport-solar-panels-safety-issues.cnn.
[2] Ho, C.K., C.M. Ghanbari and R.B. Diver, 2011, Methodology to Assess Potential Glint and Glare Hazards From Concentrating Solar Power Plants: Analytical Models and Experimental Validation, Journal of Solar Energy Engineering-Transactions of the Asme, 133(3).
[3] Chiabrando, R., E. Fabrizio and G. Garnero, 2009, The territorial and landscape impacts of photovoltaic systems: Definition of impacts and assessment of the glare risk, Renewable & Sustainable Energy Reviews, 13(9), p. 2441-2451.
[4] Ho, C.K. and C.A. Sims, 2012, Solar Glare Hazard Analysis Tool (SGHAT) User’s Manual v. 1.0, Sandia National Laboratories, SAND2012-10761P, Albuquerque, NM., sandia.gov/glare.
[5] Saur, R.L. and S.M. Dobrash, 1969, Duration of Afterimage Disability after Viewing Simulated Sun Reflections, Applied Optics, 8(9), p. 1799-1801.
[6] ABC News 20/20, 1999, Sun Glare – Sight Unseen, Charles Gibson and Michael Guillen, aired March 22, 1999.
[7] Nakagawara, V.B., K.J. Wood and R.W. Montgomery, 2003, Natural Sunlight and Its Association to Aviation Accidents: Frequency and Prevention, DOT/FAA/AM-03/6, Civil Aerospace Medical Insti- tute, Federal Aviation Administration, Oklahoma City, Okla.
8] U.S. Department of Energy EERE Website. Examples of Codes that Address Glare from Solar Energy Systems. July 30, 2012; www4.eere.energy.gov/solar/sunshot/resource_center/ask/question/question_11.
[9] Aslam, T.M., D. Haider and I.J. Murray, 2007, Principles of disability glare measurement: an ophthalmological perspective, Acta Ophthalmologica Scandinavica, 85(4), p. 354-360.
[10] Babizhayev, M.A., 2003, Glare disability and driving safety, Ophthalmic Research, 35(1), p. 19-25. [11] Bhise, V. and S. Sethumadhavan, 2008, Effect
of Windshield Veiling Glare on Driver Visibility, Transportation Research Record, (2056), p. 1-8.
[12] Osterhaus, W.K.E., 2005, Discomfort glare assessment and prevention for daylight applications in office environments, Solar Energy, 79(2), p. 140-158.
[13] Sliney, D.H. and B.C. Freasier, 1973, Evaluation of Optical Radiation Hazards, Applied Optics, 12(1), p. 1-24.