By Amory B. Lovins September 22, 2023
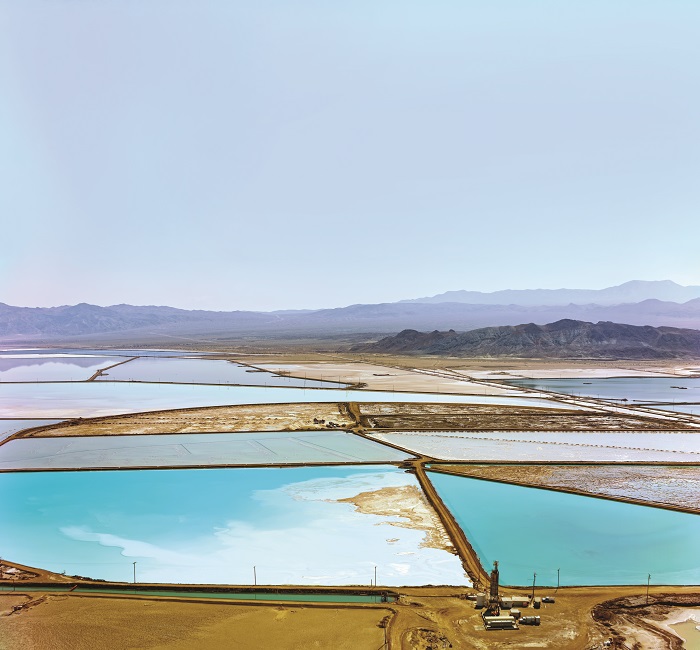
This aerial view shows the lithium mine in Silver Peak, Nevada. (Credit: simonkr)
A flood of recent articles, whether spontaneous or coordinated, seeks to discredit renewable energy, electric vehicles (EVs), and other elements of the climate-saving energy transition. Critiques range from grid reliability to land use, from economy to equity.
Among the most widespread and conflictual claims is that it’s immensely destructive, if not impossible, to find enough minerals to make all the batteries that a global fleet of EVs will need.
These mineral concerns are indeed not trivial, but are often exaggerated. I’ll outline here how they can become manageable if we include solutions often overlooked.
Battery materials like lithium, nickel and cobalt are a special case of a broader dynamic. When a mined material is expected to become scarce, its price rises. That signal elicits more-efficient use, recycling, substitution, exploration, innovation and other market responses, as I’ve described for rare earths.1
(Illustrating that article’s substitution thesis, the iron nitride supermagnets it mentioned a few years ago as an experimental ambition2 have now come to market;3 they contain no rare earths and theoretically could become twice as strong as the best rare-earth magnets.)
Mineral scarcity may be real or hyped — for example, to reduce electric vehicles’ competition with oil or to raise commodity or mining-stock prices for speculators. Some minerals may raise legitimate concerns besides scarcity, such as child labor, corruption and other abuses in artisanal cobalt mining; undue dependence on Chinese ores and processing plants; or the water use and environmental damage of mining.
Real concerns also may need context — like a recent remark, whose validity depends on many assumptions, that growing California almonds takes six times as much water per pound as mining lithium in the desert. Almonds, too, can be enjoyed just once, but once extracted, lithium can keep providing benefits more or less permanently.
And of course, renewably powered EVs displace oil-burning vehicles that importantly harm land, air, health and climate.
While there are proper concerns about mining battery minerals, there are also many powerful and multiplicative solutions that conventional projections often understate or ignore, exaggerating future mining needs. Let’s now explore six successive and multiplicative parts of the solution space.
1. Storing More Energy per Kilogram
Improving batteries’ composition, manufacturing, design, controls and recharging can store far more energy per unit of materials. Since 2010, lithium-ion battery cells have nearly tripled their energy storage per kilogram.4
Their 89% price drop over the same decade is due partly to their more-frugal use of materials. Further major gains are expected in this decade; as one of many examples, silicon anodes are said to raise lithium-ion batteries’ energy density by 20%.5
RMI assesses that technologies collectively doubling lithium-ion batteries’ energy density could enter production by 2025.6 Tesla’s 2020 Battery Day presentation announced major improvements scaling up for mass production in 2022.7 So projections based on old energy densities substantially overstate needed mining.
2. Lasting Longer, then Being “Reincarnated”
Batteries are also lasting longer as designs, materials, manufacturing and use improve. Just a new charging profile can reverse life-reducing lithium migration.8 Million-mile batteries are emerging, so their lifetime could soon become as irrelevant an issue as the speed of your modem. The longer batteries last, the more vehicle-miles their materials can support.
When an EV ultimately retires (or crashes), its battery pack can be “reincarnated” into valuable stationary storage that continues to provide great value, not by moving a vehicle but by supporting the global shift to renewable power (hence reducing fossil fuels’ mining and emissions).
Thus The Mobility House GmBH in Zürich already earns ~€1000 per EV battery pack per year by selling ~13 of 21 potential services from stationary or parked EV battery packs to the electricity grid in several European countries.9 (For example, in 2018, the firm licensed an EV as Germany’s first power plant on wheels, able to sell frequency stabilization services to the grid.)
Coordinating the immense storage and other capabilities in the world’s EVs, parked ~95% of the time and often rechargeable at flexible times, is emerging as a major and lucrative enabler of rapid growth in variable renewables — solar photovoltaics and wind power.
Utility-scale and behind-the-meter storage will compete not just with each other but also with grid-integrated, EV-based electricity storage. That and eight other kinds of carbon-free grid flexibility resources mean that utility-scale batteries are useful but not essential10 for keeping the grid reliable11 as it becomes renewable (another conversation).12
Thus, batteries for EVs and for grids are not additive requirements but complementary, shared and often successive uses of the same materials, reducing total mining needs.

3. Recycling Batteries
Recycled lithium battery cells are about 17 times richer sources of nickel, four to five times richer sources of lithium, and 10 times richer sources of cobalt than their respective natural ores. “Mining” that recycling resource is already getting well underway.13
I recently visited Tesla co-founder J.B. Straubel’s Redwood Materials plant14 in Carson City, Nevada — the leading U.S. battery recycler and an emergent world leader. The plant recycles several semi truck-loads a day of immensely diverse batteries — all kinds, shapes, sizes and uses, often collecting them from major retailers who get them from customers.
The plant converts all those batteries, with typically over 90% efficiency, into pure materials that go right back into new batteries.
In effect, Redwood Materials is a benign, non-polluting, nearly-zero-emissions “mine” producing lithium, nickel, cobalt, copper and graphite, with more products to come. By brilliant design, it produces no wastes — only value. For now, it uses a little natural gas to start several days of continuous processing fueled by electrolytes and self-sustaining reactions. Future processes will wring out that gas too and capture solid carbon.
The processing already makes money on a cash-flow basis even as capacity rapidly scales up, with 20,000 input tons per year expected in 2021. Even by May 2021, the plant was expected to recover enough battery materials each year to build 45,000 EV packs. The firm’s $0.7 billion capital raise in July 2021 was oversubscribed.
On Sept. 14, 2021, Redwood Materials announced plans for a factory to make advanced battery electrodes,15 increasingly from recycled materials — enough for about a million EVs a year by 2025, then quintupling by 2030. A week later, Ford Motor Company announced an expansive alliance to develop a closed-loop North American battery supply chain.
A major source of recyclable batteries for Redwood Materials is the Tesla Gigafactory a half-hour’s drive away — another of Straubel’s designs. It sends two truckloads a day of defective output and scrap and takes back recycled materials to make more batteries.
The two plants are symbiotic, like a lichen. Other big battery factories going up around the world will organically gain similar loop-closing partners. Much larger but later (for cars, often at least a decade later) materials recovery will come from batteries sold and used.
As more energy-dense batteries used in more-efficient EVs compete with rising EV market share, such recycling operations can already supply on the order of a tenth of the materials needed for the global EV fleet.
In time, recycling can ultimately scale to achieve steady state, eliminating further mining, at a very large industry capacity on the order of (very roughly) 10 TWh/yr — as lagged recovery catches up with saturating global EV growth over several decades. This loop-closing could about halve EVs’ total CO2 emissions.
On similar principles, Apple aims to make iPhones by 2030 that need no mining.
An analogous proof-of-concept, in a battery system that’s already around market saturation, is that about two-thirds of the world’s neurotoxic lead16 and 99% of battery lead is already recycled (about half properly, half informally and dangerously).17, 18
In almost every U.S. state, you can’t buy a lead-acid automotive battery without turning in your old one, so that loop is already very nearly closed, and lead is now rarely mined. Now Redwood Materials and its competitors aim to “mine” roughly a billion used batteries sitting unused in U.S. homes’ old laptops, cell phones, etc. — batteries whose metals are usually more valuable than lead and often rich in cobalt.
As batteries’ compositions shift, recycled streams don’t translate directly into identical battery capacity. Thus, smartphone batteries generally have high cobalt content while automotive battery makers are rapidly reducing cobalt content, so recycling smartphone batteries into EV batteries leverages ~30x more battery capacity per gram of cobalt.
To make an EV battery pack thus takes on the order of 10,000 smartphone batteries for lithium but only ~300 for cobalt. Tesla, among others, plans to eliminate its batteries’ cobalt use, but makers who still need cobalt will be able to get it from old smartphones, not child miners in the Democratic Republic of the Congo.
4. Using Novel Battery Chemistries
Several firms have demonstrated novel electrolytes that permit chemistries like rechargeable alkalines.19
Such chemistries, like manganese-zinc or manganese-aluminum, need no materials that are scarce, costly, toxic or flammable. They could thus displace lithium and nickel and cobalt, disadvantaging producers of lithium-ion batteries (notably in China).
While that lithium-ion-battery value chain displays some aspects of “lock-in,” India’s national battery mission emphasizes new chemistries20, 21, 22 (India also happens to be rich in manganese and zinc), and like other efforts elsewhere, may offer distinctive advantages that could diversify battery chemistries.
Some battery metals, like iron and aluminum, are among the most abundant elements in the Earth’s crust. Novel electrolytes could also enable safe lithium-ion and lithium-sulfur batteries suitable even for aviation.23
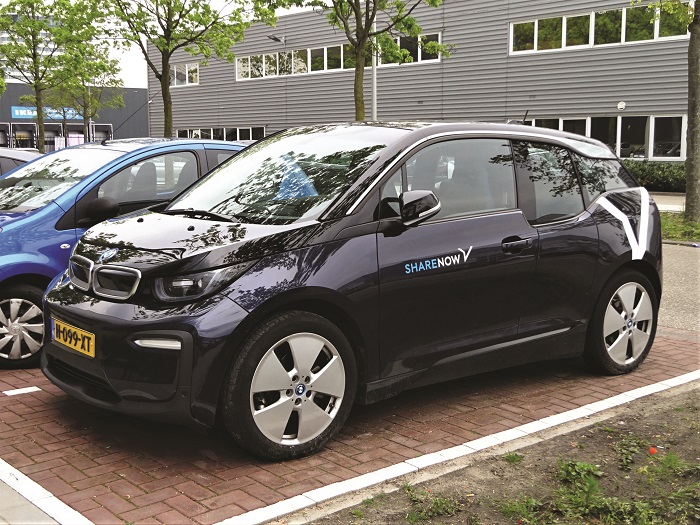
5. Introducing Efficient Vehicles
A major variable overlooked by nearly all analysts is the efficiency of the vehicle that’s being electrified. Advantageous reductions in vehicle mass, aerodynamic drag and rolling resistance — improvements in the physics of the vehicle rather than the efficiency of its electric powertrain — can cut required battery capacity for the same driving range by 2–3-fold.
BMW’s 2013–22 i3, for example, paid for its ultralight carbon-fiber body by needing fewer batteries to move less mass and by having simpler manufacturing24 (with one-third the normal investment and water and half the normal energy, space and time).
Projected battery capacity per vehicle is therefore not a fixed number but should be parameterized to platform efficiency. What is that uncounted variable’s potential range? In September 2021, 2–3-fold — and imminently severalfold more!
That’s because a further ~2–4-fold efficiency gain is being demonstrated by a new generation of vehicles, finishing their capital raise for mass production. They are so efficient that they can power a normal commuting cycle just by having solar cells on their upper surfaces.
(Disclosure: I advise two such firms — Aptera Motors at 343 miles per gallon equivalent (mpge) with two seats25 and Lightyear at 251 mpge with five.)26
Both designs can improve further. Such vehicles need proportionately smaller batteries and less or no recharging infrastructure. In round numbers, they’re 2–3-fold more efficient than, say, a Tesla Model 3, one of the most efficient EVs on the market.
Together, these efficiency gains can use batteries up to an order of magnitude (roughly a factor of 10) more efficiently than many EVs now on the market and could reduce their battery needs correspondingly, all with uncompromised safety and attractive driver attributes.
The Aptera Motors NeverCharge is a niche vehicle, but Dutch firm Lightyear’s is mainstream. Both are important, and there will be more.
6. Creating Efficient Mobility
Beyond the system boundary of the vehicle itself, productive use of vehicles, new mobility business models, virtual mobility (send electrons, leave heavy nuclei at home), and better urban design and public policy to provide better access with less driving can all dramatically affect future needs for autos and driving.
For example, the journalist Sam Deutsch reports that “Atlanta and Barcelona have a similar number of people and length of rapid transit, but Barcelona’s carbon emissions are 83% lower and mass transit ridership is 565% higher.”27
As my 2017 analysis found for rare earths,28 and the same is now true for battery minerals:
“… the most effective substitute… in both motors and batteries, isn’t another exotic material for making motors or batteries; it’s smarter car design that makes motors smaller and batteries fewer. Or, even better, it could be new business models — shareable services like Zipcar and Getaround, mobility-as-a-service operations like Lyft and Uber, or autonomous vehicles — that carry more people more miles in far fewer cars at astonishingly lower cost,29 ultimately saving on the order of $10 trillion worldwide (in net present value).”
These options span a wide range of potentially avoided vehicles, but already in some urban cores, ride-hailing services are displacing several times as many vehicles as they use. With ~4–5% average utilization of private U.S. cars, the potential is clearly far larger.
Combine that with other opportunities (with widely varying timescales and likelihoods) — ~2-fold short-term gains in battery energy density, severalfold in battery life, ~2–8+-fold in vehicle efficiency, and potentially complete displacement of scarce materials in battery chemistry — and high forecasts of demand for mined battery materials look highly uncertain and potentially wrong by large factors.
Conclusion
We have even more ways to save battery materials of concern than to increase their supply, but these demand-side opportunities are widely ignored.
Competing or comparing all options — in a whole-system perspective that emphasizes demand levers as much as supply expansions and compares or competes them — will yield better choices, actions and impacts and help to avoid asset bubbles, overbuilt supply, needless interventions and unnecessary risks.
That’s why discussions of battery materials or any other supposedly scarce resource must consider not just simplistic demand projections or worrisome mines but the whole system — end-to-end, linear-to-circular, and fully engaged with innovation, economics and trade.
This article was originally published by RMI in 2021. It was reprinted and lightly edited with permission from the author.
Sources
- https://tinyurl.com/4f9pzz44
- https://tinyurl.com/2k84j5en
- https://nironmagnetics.com/
- https://tinyurl.com/kpse7863
- https://silanano.com/
- https://tinyurl.com/3ka5fd5w
- https://tinyurl.com/34kpzznb
- https://tinyurl.com/m85bhr4w
- https://tinyurl.com/479hj6nk
- https://tinyurl.com/4f5s5dz2
- https://tinyurl.com/yck2c974
- https://tinyurl.com/3cx5b5n2
- https://tinyurl.com/s8ts3f94
- https://tinyurl.com/bdftuz6d
- https://tinyurl.com/4tn8sm2h
- https://tinyurl.com/3ewvkz2r
- https://tinyurl.com/48evjpj8
- https://tinyurl.com/5fbhhrpr
- https://ionicmaterials.com/
- https://tinyurl.com/murdkwre
- https://tinyurl.com/3fa9tmtc
- https://tinyurl.com/3ka5fd5w
- Ibid.
- https://tinyurl.com/57hjjher
- https://aptera.us/
- https://lightyear.one/
- https://tinyurl.com/5bptjrzy
- https://tinyurl.com/4f9pzz44
- https://tinyurl.com/mr3ny8ny
About the Author
Physicist Amory B. Lovins is co-founder and chairman emeritus of RMI and an adjunct professor of civil and environmental engineering at Stanford University.
Excellent and timely, as usual for Emory. ASES should publish more of such articles and not wait so long. One related issue on mining for such minerals as with the Alaska mining road fight (under debate with the Biden Administration) is that the news articles always blame the clean energy revolution for the need of such minerals, NEVER discussing the mineral needs of the fossil fuel industry, which I would assume is greater than clean energy. There is a lot of imbedded and needed copper, lead, lithium, etc. in the fossil and other industries. Tear down the fossil industry and recycle those oil rigs into wind turbines and solar arrays, making clean energy cheaper than drilling a new oil and gas well. Lots of available minerals!!